Cardiac output in a normal individual at rest ranges between 4 to 6 liters per minute, but during
severe exercise the heart may be required to pump three to four times this amount. There are two
primary modes by which the blood volume pumped by the heart, at any given moment, is regulated: 1)
intrinsic cardiac regulation, in response to changes in the volume of blood flowing into the heart;
and 2) control of heart rate and cardiac contractility by the autonomic nervous system.
The intrinsic ability of the heart to adapt to changing volumes of inflowing blood is known as
the Frank-Starling mechanism (law) of the heart. In general, this response can simply be described
as: the more the heart is stretched (increased blood volume), the greater will be the subsequent
force of ventricular contraction and, thus, the amount of blood ejected through the aortic valve.
In other words, within its physiological limits, the heart will pump out all the blood that enters
it without allowing excessive damming of blood in veins. The underlying basis for this phenomenon
is related to the optimization of the lengths of sarcomeres and the functional subunits of striate
muscle; there is optimization in the potential for the contractile proteins (actin and myosin) to
form crossbridges. It should also be noted that "stretch" of the right atrial wall (e.g., due to
increased venous return) can directly increase the rate of the sinoatrial node by 10-20%; this also
aids in the amount of blood that will ultimately be pumped per minute by the heart.
The pumping effectiveness of the heart is also effectively controlled by the autonomic nervous
system by both the sympathetic and parasympathetic components of this system. There is extensive
innervation of the myocardium by such. To get a feel for how effective the modulation of the heart
by this innervation is, it has been reported that the cardiac output often can be increased by more
than 100% by sympathetic stimulation and, by contrast, output can be nearly terminated by
parasympathetic (vagal) stimulation.
Cardiovascular function is also modulated through reflex mechanisms that involve baroreceptors,
the chemical composition of the blood, and via the release of various hormones. More specifically,
baroreceptors, which are located in the walls of some arteries and veins, exist to monitor the
relative blood pressure. Those specifically located in the carotid sinus help to reflexively
maintain normal blood pressure in the brain, whereas those located in the area of the ascending arch
of the aorta help to govern general systemic blood pressure (for more details, see the chapter on
the Autonomic Nervous System). Chemoreceptors that monitor the chemical composition of blood are
located close to the baroreceptors of the carotid sinus and arch of the aorta, in small structures
known as the carotid and aortic bodies. The chemoreceptors within these bodies detect changes in
blood levels of O2, CO2, and H+. Hypoxia (a low availability of O2), acidosis (increased blood
concentrations of H+), and/or hypercapnia (high concentrations of CO2) stimulate the chemoreceptors
to increase their action potential firing frequencies to the brain cardiovascular control centers.
In response to this increased signaling, the central nervous system control centers, the
hypothalamus, in turn, cause an increased sympathetic stimulation to arterioles and veins, producing
vasoconstriction and a subsequent increase in blood pressure. In addition, the chemoreceptors
simultaneously send neural input to the respiratory control centers in the brain, so to induce the
appropriate control of respiratory function (e.g., increase O2 supply and reduce CO2 levels
The overall functional arrangement of the blood circulatory system is shown in Figure 6. The
role of the heart needs be considered in three different ways: as the right pump, as the left pump,
and as the heart muscle tissue which has its own metabolic and flow requirements. As described
above, the pulmonary (right heart) and system (left heart) circulations are arranged in a series.
Thus, cardiac output increases in each at the same rate; hence an increased systemic need for a
greater cardiac output will automatically lead to a greater flow of blood through the lungs (a
greater potential for O2 delivery). In contrast, the systemic organs are functionally arranged in a
parallel arrangement; hence: 1) nearly all systemic organs receive blood with an identical
composition (arterial blood); and 2) the flow through each organ can be and is controlled
independently.
For example, during exercise, the circulatory response is an increase in blood flow through some
organs (e.g., heart, skeletal muscle, brain) but not others (e.g., kidney and gastrointestinal
system). The brain, heart and skeletal muscles typify organs in which blood flows solely to supply
the metabolic needs of the tissue; they do not recondition the blood. The blood flow to the heart
and brain is normally only slightly greater than that required for their metabolism; hence small
interruptions in flow are not well tolerated. For example, if coronary flow to the heart is
interrupted, electrical and/or functional (pumping ability) activities will noticeable be altered
within a few beats. Likewise, stoppage of flow to the brain will lead to unconsciousness within a
few seconds and permanent brain damage can occur in as little as four minutes without flow. The
flow to skeletal muscles can dramatically change (flow can increase from 20-70% of total cardiac
output) depending on use and thus their metabolic demand.
Many organs in the body perform the task of continually reconditioning the circulating blood.
Primary organs performing such tasks include: 1) the lungs (O2 and CO2 exchange); 2) the kidneys
(blood volume and electrolyte composition, Na+, K+, Ca2+, Cl- and phosphate ions); and 3) the skin
(temperature). Blood conditioning organs can often withstand, for short periods of time,
significant reductions of blood flow without subsequent compromise.
|
|
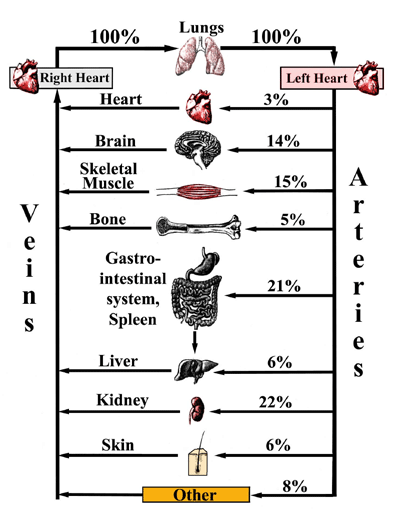
Figure 6. Provided is a functional representation of the blood circulatory system. The percentages
indicate the approximate relative percentages of the cardiac output that is delivered, at a given
moment in time, to the major organ systems within the body.
|
|
|